3.1 Origin of Earth and the Solar System
Modified from Karla Panchuk in "Physical Geology" by Steven Earle*
According to the Big Bang theory, the universe blinked violently into existence 13.77 billion years ago (Figure 3.1.1). The Big Bang is often described as an explosion, but imagining it as an enormous fireball isn’t accurate. The Big Bang involved a sudden expansion of matter, energy, and space from a single point. The kind of Hollywood explosion that might come to mind involves expansion of matter and energy within space, but during the big bang, space itself was created.
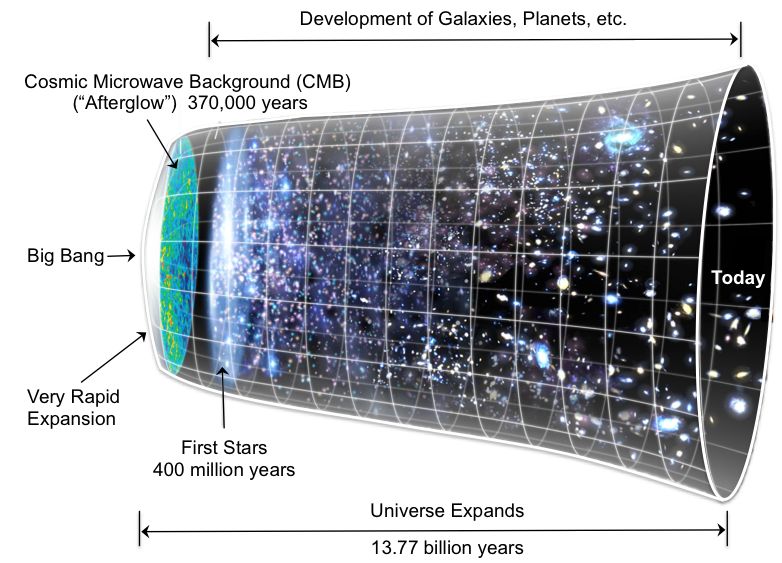
At the start of the Big Bang, the universe was too hot and dense to be anything but a sizzle of particles smaller than atoms, but as it expanded, it also cooled. Eventually some of the particles collided and stuck together. Those collisions produced hydrogen and helium, the most common elements in the universe, along with a small amount of lithium. Gravity caused clouds of these early elements to coalesce into stars, and it was inside these stars that heavier elements were formed
Our solar system began to form around 5 billion years ago, roughly 8.7 billion years after the Big Bang. A solar system consists of a collection of objects orbiting one or more central stars. All solar systems start out the same way. They begin in a cloud of gas and dust called a nebula. Nebulae are some of the most beautiful objects that have been photographed in space, with vibrant colors from the gases and dust they contain, and brilliant twinkling from the many stars that have formed within them (Figure 3.1.2). The gas consists largely of hydrogen and helium, and the dust consists of tiny mineral grains, ice crystals, and organic particles.
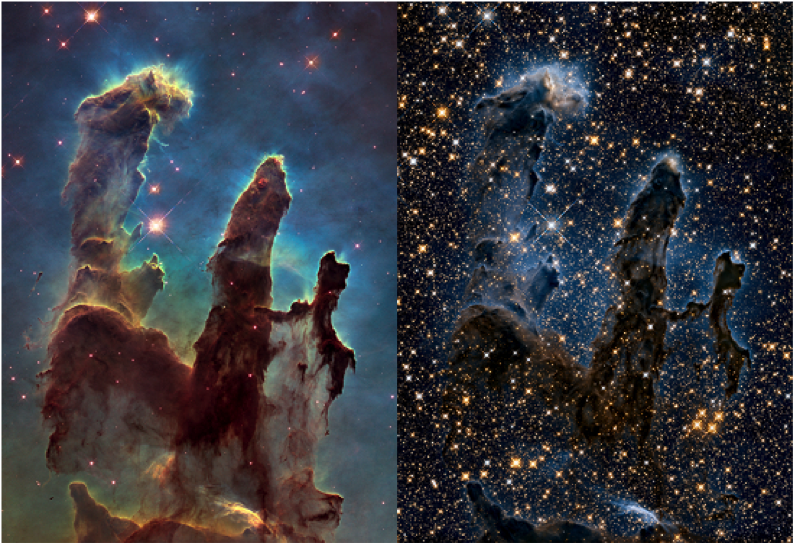
A solar system begins to form when a small patch within a nebula (small by the standards of the universe, that is) begins to collapse upon itself. Exactly how this starts isn’t clear, although it might be triggered by the violent behavior of nearby stars as they progress through their life cycles. Energy and matter released by these stars might compress the gas and dust in nearby neighborhoods within the nebula. Once it is triggered, the collapse of gas and dust within that patch continues for two reasons. One of those reasons is that gravitational force pulls gas molecules and dust particles together. But early in the process, those particles are very small, so the gravitational force between them isn’t strong. So how do they come together? The answer is that dust first accumulates in loose clumps for the same reason dust bunnies form under your bed: static electricity. As the small patch within a nebula condenses, a star begins to form from material drawn into the center of the patch, and the remaining dust and gas settle into a disk that rotates around the star. The disk is where planets eventually form, so it’s called a protoplanetary disk. In Figure 3.1.3 the image in the upper left shows an artist’s impression of a protoplanetary disk, and the image in the upper right shows an actual protoplanetary disk surrounding the star HL Tauri. Notice the dark rings in the protoplanetary disk. These are gaps where planets are beginning to form. The rings are there because incipient planets are beginning to collect the dust and gas in their orbits. There is an analogy for this in our own solar system, because the dark rings are akin to the gaps in the rings of Saturn (Fig. 3.1.3, lower left), where moons can be found (Fig. 3.1.3, lower right).
![Figure 3.1.3 Protoplanetary disks and Saturn’s rings. Upper left: An artists impression of a protoplanetary disk containing gas and dust, surrounding a new star. [NASA/ JPL-Caltech, http://1.usa.gov/1E5tFJR] Upper right: A photograph of the protoplanetary disk surrounding HL Tauri. The dark rings within the disk are thought to be gaps where newly forming planets are sweeping up dust and gas. [ALMA (ESO/NAOJ/NRAO) http://bit.ly/1KNCq0e]. Lower left: A photograph of Saturn showing similar gaps within its rings. The bright spot at the bottom is an aurora, similar to the northern lights on Earth. [NASA, ESA, J. Clarke (Boston University), and Z. Levay (STScI) http://bit.ly/1IfSCX5] Lower right: a close-up view of a gap in Saturn’s rings showing a small moon as a white dot. [NASA/JPL/Space Science Institute, http://1.usa.gov/1g2EeYw]](https://rwu.pressbooks.pub/app/uploads/sites/7/2019/05/figure3.1.3-2.png)
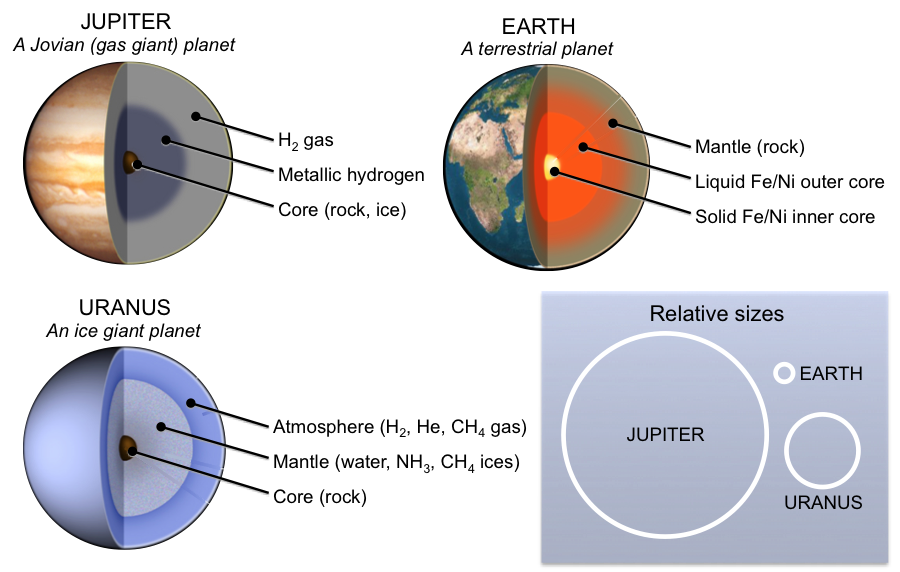
These three types of planets are not mixed together randomly within our solar system. Instead they occur in a systematic way, with terrestrial planets closest to the sun, followed by the Jovian planets and then the ice giants. Part of the reason for this arrangement is the frost line (also referred to as the snow line). The frost line separated the inner part of the protoplanetary disk closer to the sun, where it was too hot to permit anything but silicate minerals and metal to crystallize, from the outer part of the disk farther from the Sun, where it was cool enough to allow ice to form. As a result, the objects that formed in the inner part of the protoplanetary disk consist largely of rock and metal, while the objects that formed in the outer part consist largely of gas and ice. The young sun also blasted the solar system with raging solar winds (winds made up of energetic particles), which helped to drive lighter molecules toward the outer part of the protoplanetary disk.
The objects in our solar system formed by accretion. Early in this process, mineral and rock particles collected in fluffy clumps because of static electricity. As the mass of the clumps increased, gravity became more important, pulling material from farther away and growing these solid masses into larger and larger bodies. Eventually the mass of the objects became large enough that their gravity was strong enough to hang onto gas molecules, because gas molecules are very light.
Our Earth formed though this process of accretion about 4.6 billion years ago. The early Earth was very hot and had a molten, fluid composition, with lost of geological and volcanic activity on the surface. The Earth’s heat came from a variety of processes:
- Heat came from the decay of radioactive elements within the Earth, specifically the decay of 235U, 238U, 40K, and 232Th, which are primarily present in the mantle. The total heat produced that way has been decreasing over time (because these isotopes are getting used up), and is now roughly 25% of what it was when Earth formed. This means that Earth’s interior is slowly becoming cooler.
- Heat came from the thermal energy already contained within the objects that accreted to form the Earth.
- Heat came from collisions. When objects hit Earth, some of the energy from their motion went into deforming Earth, and some of it was transformed into heat. (The very worst collision that Earth experienced was with a planet named Theia, which was approximately the size of Mars. Not long after Earth formed, Theia struck Earth. When Theia slammed into Earth, Theia’s metal core merged with Earth’s core, and debris from the outer silicate layers was cast into space, forming a ring of rubble around Earth. The material within the ring coalesced into a new body in orbit around Earth, giving us our moon. Remarkably, the debris may have coalesced in 10 years or fewer! This scenario for the formation of the moon is called the giant impact hypothesis.)
- As Earth became larger, its gravitational force became stronger. This increased Earth’s ability to draw objects to it, but it also caused the material making Earth to be compressed, rather like Earth giving itself a giant gravitational hug. Compression causes materials to heat up.
Heating had a very important consequence for Earth’s structure. As Earth grew, it collected a mixture of silicate mineral grains as well as iron and nickel. These materials were scattered throughout Earth. That changed when Earth began to heat up: it got so hot that both the silicate minerals and the metals melted. The metal melt was much denser than the silicate mineral melt, so the metal melt sank to Earth’s center to become its core, and the silicate melt rose upward to become Earth’s crust and mantle. In other words, Earth unmixed itself. The separation of silicate minerals and metals into a rocky outer layer and a metallic core, respectively, is called differentiation. Gravity has since pulled Earth into an almost spherical shape with a radius of 6371 km, and a circumference of about 40,000 km. However, it is not a perfect sphere, as the Earth’s rotation causes an equatorial bulge, so that the Earth’s circumference is 21 km (0.3%) wider at the equator than it is pole to pole. Thus it is technically an “oblate spheroid.”
If we were to take an inventory of the elements that make up Earth, we would find that 95% of Earth’s mass comes from only four elements: oxygen, magnesium, silicon, and iron. Most of the remaining 5% comes from aluminum, calcium, nickel, hydrogen, and sulphur. We know that the Big Bang made hydrogen, helium, and lithium, but where did the rest of the elements come from? The answer is that the other elements were made by stars. The heat and pressure within stars cause smaller atoms to smash together and fuse into new, larger atoms. For example, when hydrogen atoms smash together and fuse, helium is formed. Large amounts of energy are released when some atoms fuse and that energy is what causes stars to shine.
It takes larger stars to make elements as heavy as iron and nickel. Our Sun is an average star; after it uses up its hydrogen fuel to make helium, and then some of that helium is fused to make small amounts of beryllium, carbon, nitrogen, oxygen, and fluorine, it will be at the end of its life. It will stop making atoms and will cool down and bloat until its middle reaches the orbit of Mars. In contrast, large stars end their lives in spectacular fashion, exploding as supernovae and casting off newly formed atoms —including the elements heavier than iron — into space. It took many generations of stars creating heavier elements and casting them into space before heavier elements were abundant enough to form planets like Earth.
*”Physical Geology” by Steven Earle used under a CC-BY 4.0 international license. Download this book for free at http://open.bccampus.ca
the theory that the universe started with a giant expansion approximately 13.77 billion years ago (3.1)
a star and the planets surrounding it (3.1)
a cloud of interstellar dust and gases (3.1)
a rotating cloud of gas and dust surrounding a young star (3.1)
a planet with a rocky mantle and crust and metallic core (e.g., Earth) (3.1)
a gas giant (3.1)
a large planet composed mostly of hydrogen and helium (e.g. Jupiter) (3.1)
a planet that is comprised mainly of gases heavier than hydrogen and helium, including oxygen, carbon, nitrogen, and sulfur (e.g., Uranus and Neptune) (3.1)
in the context of planetary systems the boundary beyond which volatile components (e.g., water, carbon dioxide, methane, ammonia etc.) are frozen (3.1)
in astronomy the radius around a star at which represents the boundary between gases (or liquids) and solids (3.1)
a stream of ionized (charged) particles away from the Sun (3.1)
the process by which solid celestial bodies are added to existing bodies during collisions (3.1)
the metallic interior part of the Earth, extending from a depth of 2900 km to the center (3.2)
the theory that the Moon formed when a Mars-sized planet (Theia) collided with the Earth at 4.5 billion years ago (3.1)
the uppermost layer of the Earth, ranging in thickness from about 5 km (in the oceans) to over 50 km (on the continents) (3.2)
the middle layer of the Earth, dominated by iron and magnesium rich silicate minerals and extending for about 2900 km from the base of the crust to the top of the core (3.2)
the un-mixing of a magma, typically by the physical separation of minerals that crystallize early and settle towards the bottom (3.1)