12.3 Biogenous Sediments
Biogenous sediments come from the remains of living organisms that settle out as sediment when the organisms die. It is the “hard parts” of the organisms that contribute to the sediments; things like shells, teeth or skeletal elements, as these parts are usually mineralized and are more resistant to decomposition than the fleshy “soft parts” that rapidly deteriorate after death.
Macroscopic sediments contain large remains, such as skeletons, teeth, or shells of larger organisms. This type of sediment is fairly rare over most of the ocean, as large organisms don’t die in enough of a concentrated abundance to allow these remains to accumulate. One exception is around coral reefs; here there is a great abundance of organisms that leave behind their remains, in particular the fragments of the stony skeletons of corals that make up a large percentage of tropical sand.
Microscopic sediment consists of the hard parts of microscopic organisms, particularly their shells, or tests. Although very small, these organisms are highly abundant and as they die by the billions every day their tests sink to the bottom to create biogenous sediments. Sediments composed of microscopic tests are far more abundant than sediments from macroscopic particles, and because of their small size they create fine-grained, mushy sediment layers. If the sediment layer consists of at least 30% microscopic biogenous material, it is classified as a biogenous ooze. The remainder of the sediment is often made up of clay.
The primary sources of microscopic biogenous sediments are unicellular algaes and protozoans (single-celled amoeba-like creatures) that secrete tests of either calcium carbonate (CaCO3) or silica (SiO2). Silica tests come from two main groups, the diatoms (algae) and the radiolarians (protozoans) (Figure 12.3.1).
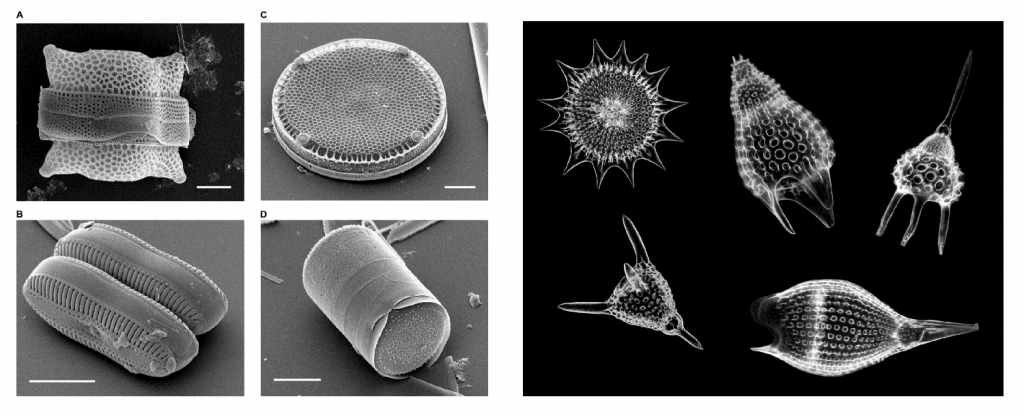
Diatoms are important members of the phytoplankton, the small, drifting algal photosynthesizers. A diatom consists of a single algal cell surrounded by an elaborate silica shell that it secretes for itself. Diatoms come in a range of shapes, from elongated, pennate forms, to round, or centric shapes that often have two halves, like a Petri dish (Figure 12.3.1 left). In areas where diatoms are abundant, the underlying sediment is rich in silica diatom tests, and is called diatomaceous earth (see box below).
What use are diatoms?
Diatoms are a vital piece of the global ecosystem for their role in oceanic primary production and the creation of much of the oxygen that organisms breathe. But diatoms are also important for many industrial and agricultural applications. Because of the very fine grain size, and the lattice-like structure of the diatom tests, diatomaceous earth has been used as a filtering agent in things like swimming pool filters and beer brewing. The microscopic tests have been added as an abrasive to toothpaste, facial cleansers and household cleaning agents. Alfred Nobel used diatomaceous earth to stabilize nitroglycerine in the production of dynamite. Diatomaceous earth also displays insecticide properties by stimulating dehydration in insects. It is marketed for this purpose in agriculture, as well as for household use to combat ants, cockroaches, and bedbugs. “Food grade” diatomaceous earth has also entered the market, with proponents touting a range of health benefits arising from its consumption. That’s a pretty impressive range of uses from a microscopic algae!
Radiolarians are planktonic protozoans (making them part of the zooplankton), that like diatoms, secrete a silica test. The test surrounds the cell and can include an array of small openings through which the radiolarian can extend an amoeba-like “arm” or pseudopod (Figure 12.3.1 right). Radiolarian tests often display a number of rays protruding from their shells which aid in buoyancy. Oozes that are dominated by diatom or radiolarian tests are called siliceous oozes.
Like the siliceous sediments, the calcium carbonate, or calcareous sediments are also produced from the tests of microscopic algae and protozoans; in this case the coccolithophores and foraminiferans. Coccolithophores are single-celled planktonic algae about 100 times smaller than diatoms. Their tests are composed of a number of interlocking CaCO3 plates (coccoliths) that form a sphere surrounding the cell (Figure 12.3.2 left). When coccolithophores die the individual plates sink out and form an ooze. Over time, the coccolithophore ooze lithifies to becomes chalk. The famous White Cliffs of Dover in England are composed of coccolithophore-rich ooze that turned into chalk deposits (Figure 12.3.2 right).
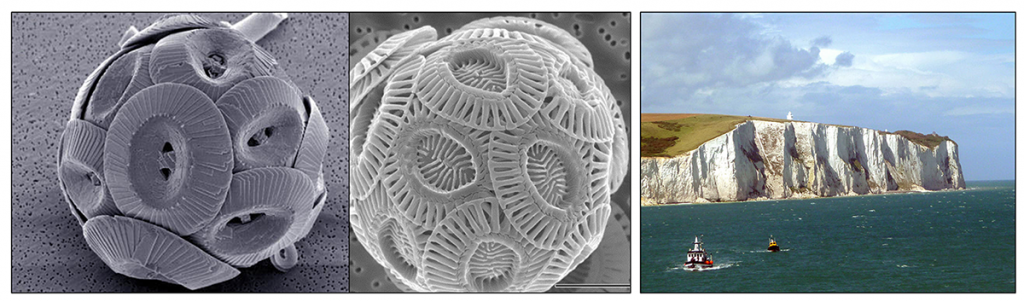
Foraminiferans (also referred to as “forams”) are protozoans whose tests are often chambered, similar to the shells of snails. As the organism grows, is secretes new, larger chambers in which to reside. Most foraminiferans are benthic, living on or in the sediment, but there are some planktonic species living higher in the water column. When coccolithophores and foraminiferans die, they form calcareous oozes.
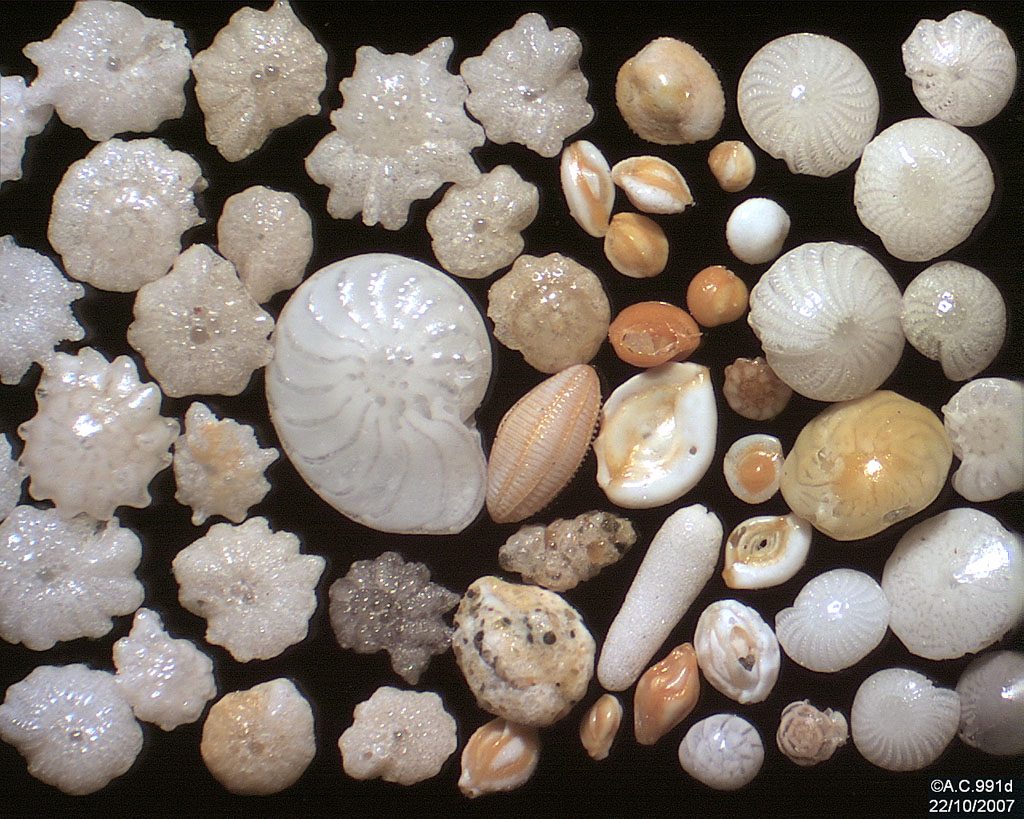
Older calcareous sediment layers contain the remains of another type of organism, the discoasters; single-celled algae related to the coccolithophores that also produced calcium carbonate tests. Discoaster tests were star-shaped, and reached sizes of 5-40 µm across (Figure 13.3.4). Discoasters went extinct approximately 2 million years ago, but their tests remain in deep, tropical sediments that predate their extinction.
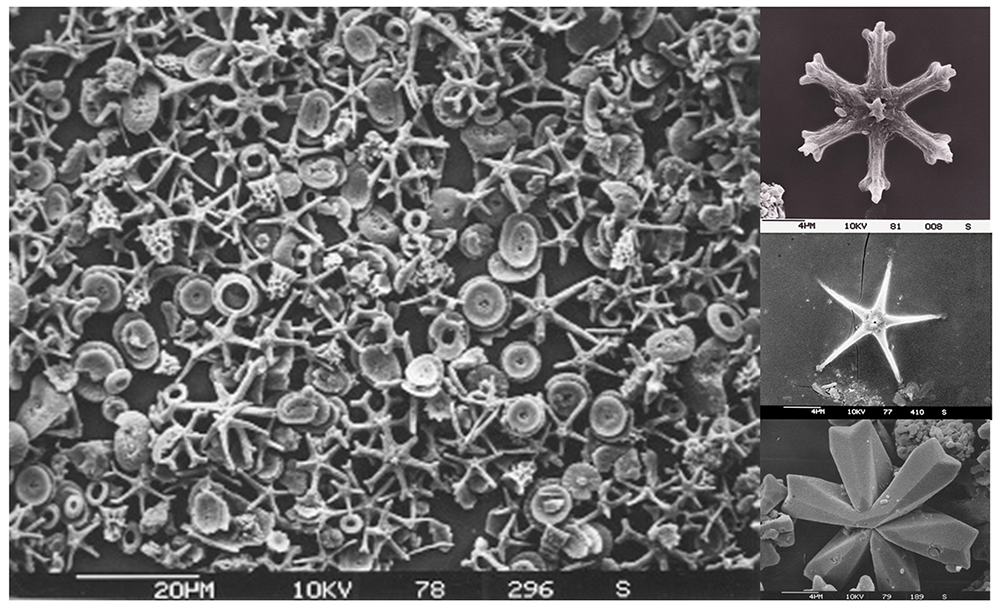
Because of their small size, these tests sink very slowly; a single microscopic test may take about 10-50 years to sink to the bottom! Given that slow descent, a current of only 1 cm/sec could carry the test as much as 15,000 km away from its point of origin before it reaches the bottom. Yet despite this, we find that the sediments in a particular location are well-matched to the types of organisms and degree of productivity that occurs in the water overhead. This means that the sediment particles must be sinking to the bottom at a much faster rate, so that they accumulate below their point of origin before the currents can disperse them. What is the mechanism for this increased sinking rate? Apparently most of the tests do not sink as individual particles; about 99% of them are first consumed by some other organism, and are then aggregated and expelled as large fecal pellets, which sink much more quickly and reach the ocean floor in only 10-15 days. This does not give the particles as much time to disperse, and the sediment below will reflect the production occurring near the surface. The increased rate of sinking through this mechanism is called the “fecal express.”
Reconstructing past climate through sediment analysis
As outlined in the opening to this chapter, examining marine sediments allows us to learn much about oceanographic and atmospheric processes, both past and present. Biogenous sediments are no exception, and they can allow us to reconstruct past climate history from oxygen isotope ratios.
Oxygen atoms exist in three forms, or isotopes, in ocean water: O16, O17 and O18 (the number refers to the atomic masses of the isotopes). O16 is the most common form, followed by O18 (O17 is rare). O16 is lighter than O18, so it evaporates more easily, leading to water vapor that has a higher proportion of O16. During periods of cooler climate, water vapor condenses into rain and snow, which forms glacial ice that has a high proportion of O16. The remaining seawater therefore has a relatively higher proportion of O18. Marine organisms who incorporate dissolved oxygen into their shells as calcium carbonate will therefore have shells with a higher proportion of O18 isotope. In other words, the ratio of O16:O18 in shells will be low during periods of colder climate.
When the climate warms, glacial ice melts, releasing O16 from the ice and returning it to the oceans, increasing the O16:O18 ratio in the water. Now, when organisms incorporate oxygen into their shells, the shells will contain a higher O16:O18 ratio. Scientists can therefore examine biogenous sediments, calculate the O16:O18 ratios for samples of known ages, and from those ratios, infer the climate conditions under which those shells were formed. The same types of measurements can also be taken from ice cores; a decrease of 1 ppm O18 between ice samples represents a decrease in temperature of 1.5o C.
sediment created from the remains of organisms (12.3)
the shell-like hard parts (either silica or carbonate) of small organisms such as radiolarians and foraminifera (12.3)
a sediment composed of >30% biogenous material (12.3)
sediment particle that is less than 1/256 mm in diameter (12.1)
photosynthetic algae that make their tests (shells) from silica (7.2)
microscopic (0.1 to 0.2 mm) marine protozoa that produce silica shells (12.3)
drifting, usually single-celled algae that undergo photosynthesis (7.1)
the production of organic compounds from carbon dioxide and water, using sunlight as an energy source (5.5)
powdery sediment composed of silica diatom tests (12.3)
small, drifting carnivorous organisms (7.1)
sediment dominated by particles of silica, often from the shells of marine organisms (7.2)
sediments composed of calcium carbonate, often from the shells of marine organisms (12.3)
photosynthetic algae that makes its test (shell) out of calcium carbonate (7.2)
the conversion of unconsolidated sediments into rock by compaction and cementation (12.1)
a single-celled protist with a shell that is typically made of calcium carbonate (12.3)
refers to the environment of the seafloor (1.3)
an organism that cannot swim effectively, so it drifts with the currents (7.1)
an extinct form of single-celled algae that produced calcareous tests that can still be found in some marine sediments (12.3)
forms of the same element that contain equal numbers of protons but different numbers of neutrons in their nuclei
parts per million